A Beginner's Guide to Injection Molding
Learn the basics to the injection molding process, including: how it works, design principles, materials, and quality systems
What is Injection Molding?
Injection molding is process tailored for producing large volumes of parts. Compared to other manufacturing technologies—like CNC machining and 3D printing—it requires an upfront, capital investment into tooling. But individual piece-part price will be substantially less when compared to other means of manufacturing plastic parts. This cost structure makes it an affordable solution for production runs.
It's most often used for the manufacturing plastic parts at scale due to its low material waste and low cost per part. It’s an ideal manufacturing process for industries like medical devices, consumer products, and automotive to name a few.
How Does Injection Molding Work?
Tooling fabrication: Once an injection molding design is finalized the first step in the manufacturing process is to mill the tooling, which is typically fabricated from steel or aluminum. In most cases, the metal block of material is placed in a CNC mill, which then carves out a negative of the final plastic part. Additional treatments like polishing or laser etching can then be applied to the tooling to achieve specific surface finishes.
Part production: The actual production of plastic parts begins by loading resin pellets into a barrel. The temperature of the barrel is raised until the resin pellets reach a molten state and are then compressed. Next, the molten plastic is injected into the metal tool through a runner system, which then feed into the mold cavity through gates. The part then cools down, solidifies, and is ejected from the tool with ejector pins.
Types of Injection Molding
The term injection molding encompasses a handful of processes that inject liquid resin into a tool to form plastic parts. Here are four common types:
Thermoplastic injection molding: Thermoplastic injection molding is the most common type of molding. It injects thermoplastic resin into the mold where the material cools to form the final part.
Liquid silicone rubber molding: Liquid silicone rubber uses thermoset materials and a chemical reaction creates the plastic part.
Overmolding: Overmolding is a process used to manufacturing plastic parts with two or more materials. You’ll often find this on parts to improve grip by adding rubber to the handle.
Insert Molding: Insert molding is process that begins with an insert component placed into the mold before resin enters. The material is then injected and flows around the insert, typically metal, to form the final part. This is frequently used for parts that require metal threads.
Basic Design Principles for Injection Molding
Tolerances
With our injection molding process, we can hold about ±0.003 in. machining accuracy. Shrink tolerance depends mainly on part design and resin choice. It varies from 0.002 in./in. for stable resins like ABS and polycarbonate to 0.025 in./in. for unstable resins like TPE.
Wall Thickness
Wall thickness is important because it can lead to defects such as sink and warp. It is best practice to maintain a uniform thickness throughout an injection-molded part. We recommend walls to be no less than 40 to 60 percent of adjacent wall thickness, and all should fit within recommended thickness ranges for the selected resin.
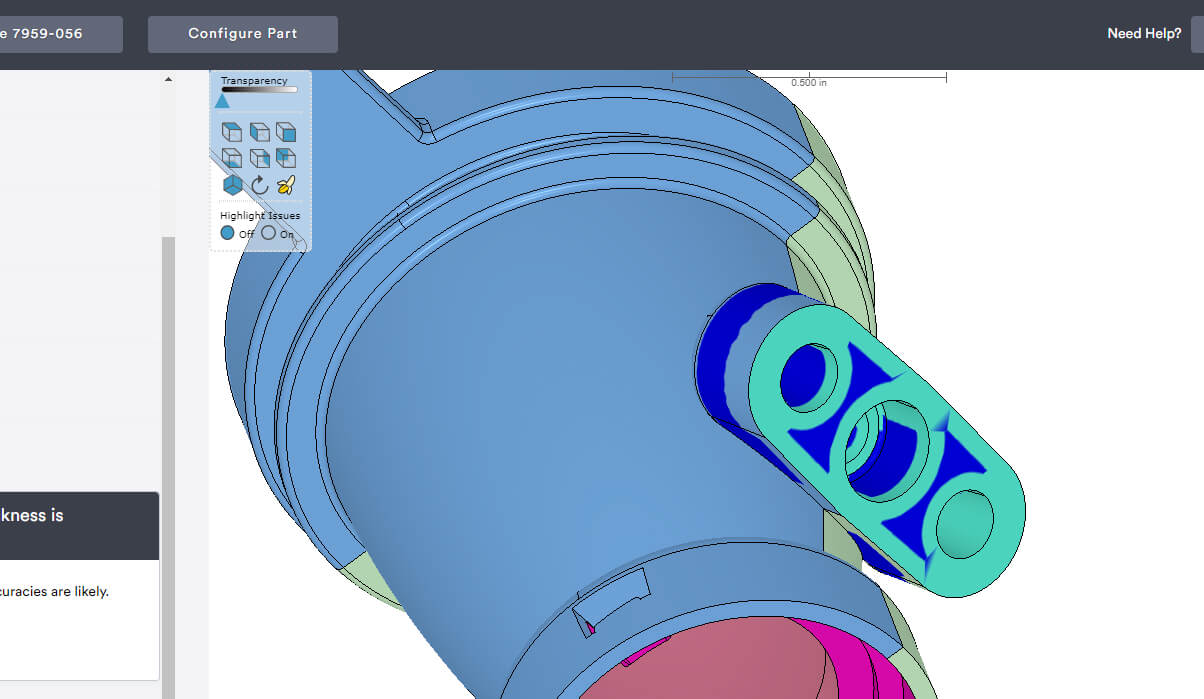
Our online quoting platform will call out features that are too thick by highlighting them in dark blue, as seen above.
Core Geometry
Core out parts to eliminate thick walls. You get the same functionality in a good molded part. Unnecessary thickness can alter part dimensions, reduce strength, and necessitate post-process machining.
Draft
Applying draft to molded parts is critical to ensure parts do not warp during the cool down process and it helps the part easily eject from the mold. Applying 1 to 2 degrees works well in most scenarios. If there are vertical faces, we advise incorporating .5 degrees of draft.
Side Actions
A portion of the mold that is pushed into place as the mold closes, using a cam-actuated slide. Typically, side-actions are used to resolve an undercut, or sometimes to allow an undrafted outside wall. As the mold opens, the side action pulls away from the part, allowing the part to be ejected. Also called a “cam.”
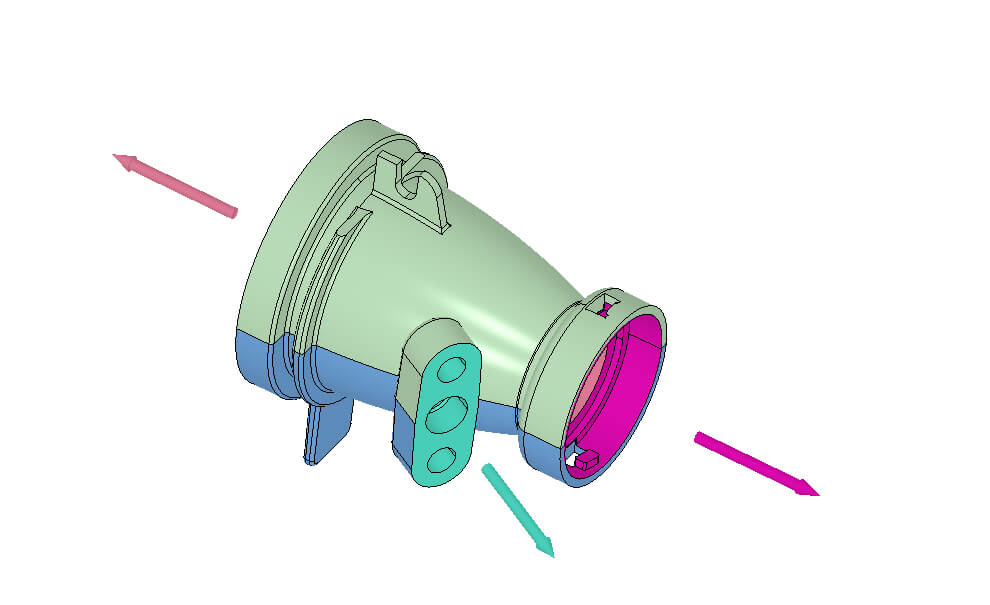
Our quoting platform identifies undercut features require side actions with solid arrows and faces with corresponding colors.
Undercuts
A portion of the part that shadows another portion of the part, creating an interlock between the part and one or both of the mold halves. An example is a hole perpendicular to the mold opening direction bored into the side of a part. An undercut prevents the part from being ejected, or the mold from opening, or both.
Bosses
A raised stud feature that is used to engage fasteners or support features of other parts passing through them. There can be a tendency to design thick bosses which will increase the likelihood of sink and voids in a part. Consider reinforcing bosses with ribs or gussets for extra strength
Gates
A gate is an opening in the injection mold tool that allows resin to enter and fill the cavity. There are three common types of injection molding gates.
- Tab gates are the most common type of gate since it works well with additives and is the most cost effective option.
- Hot Tip gate is best for parts that cosmetic appearance is a priority. These gates can also reduce wear on tooling and flash.
- Pin, Post, or Tunnel gates are ideal for cosmetic parts that don’t require a vestige. Sometimes not an option depending on material and geometry.
Ribs
A rib is a thin, wall-like feature parallel to the mold opening direction, its used to add strength and support to features like bosses and walls. To prevent sink, ribs should be no more than 60% of the wall’s thickness.
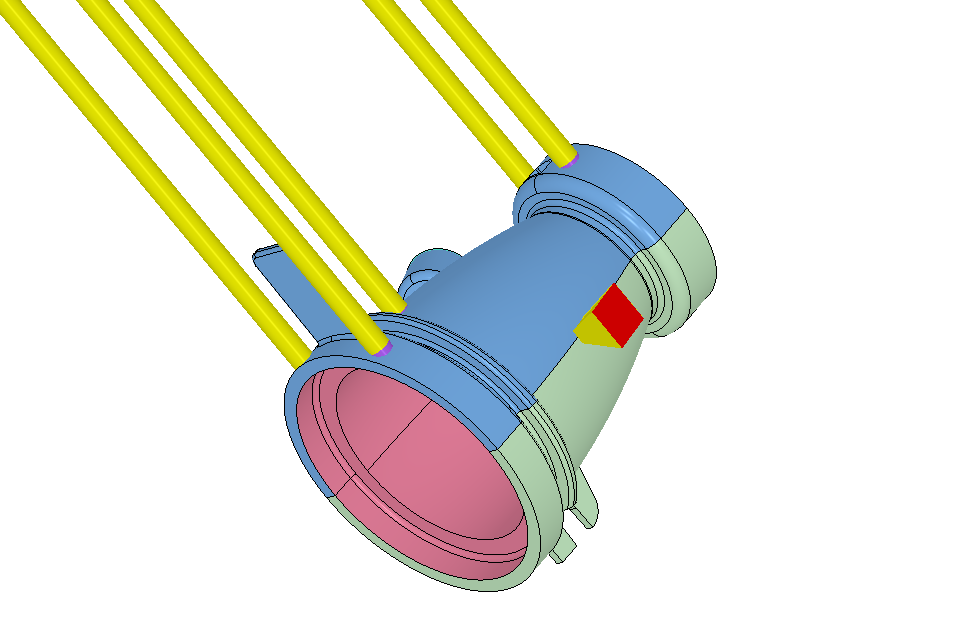
Within the 3D render of your manufacturing analysis you can also see where ejector pins and gates will be located.
Ejector Pins
Ejector pins are installed in the B-side of the mold and help to release the plastic part from the tool after the part has cooled sufficiently. Designing in sufficient draft can help reduce the need for ejector pins on a part.
Logos and Text
Sans serif fonts will be the easiest to mill into a mold with text. We recommend font larger than 20 pt. and no deeper than 0.010 in to 0.015 in.

Design for Moldability Toolkit
No one ever said that designing and manufacturing molded parts was easy. Our goal is to get you through it quickly and with quality parts. A good starting point is our Design for Moldability Toolkit.
Check Out the ToolkitInjection Molding Resins
When choosing a material for your part, relevant properties might include mechanical, physical, chemical resistance, heat, electrical, flammability or UV resistance. Resin manufacturers, compounders and independent resin search engines have data online. Here is a quick look at some common commodity and engineering resins.
Common Engineering Resins
ABS: ABS is a great choice for a vast majority of parts. It’s reasonably priced, strong, relatively tough, has a decent appearance and is forgiving even if you don’t follow all the standard design rules for plastic parts.
Acetal: A strong material with good lubricity
LCP: A very strong material that flows well, especially for thin parts. Produces weak knit lines.
Nylon: Affordable cost, strong, and wear resistant. Can be susceptible to shrink and warp, particularly glass-filled nylon.
Polycarbonate (PC): Can tolerate higher temperatures and more durable than a typical ABS but less forgiving when it comes to moldability.
PMMA (Acrylic): An affordable option for clear parts although it can be brittle.
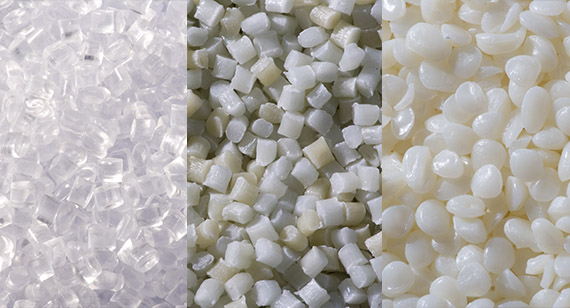
Commodity Resins
Polypropylene (PP): PP is a cheap material and good when cosmetics and rigidity aren’t a priority. Chemical resistant and good for living hinge designs.
Polyethylene: High and low density options. Is durable and chemical resistant.
Polystyrene: A hard thermoplastic that is cheap and clear.
Check out our guide to injection molding materials if you want to dig deeper into plastic selection.
Colorants and Resin Additives for Injection Molding
Stock colors from the resin vendor are typically black and natural. Natural might be white, beige, amber or another color. Semi-custom colors are created when colorant pellets are added to natural resins. For available colors, visit our materials page. There is no added charge for our inventory colors. They may not be an exact match and may create streaks or swirls in parts.
Resin Additives
Short glass fibers are used to strengthen a composite and reduce creep, especially at higher temperatures. They make the resin stronger, stiffer, and more brittle. They can cause warp due to the difference in cooling shrink between the resin and the fibers.
Carbon fiber is used to strengthen and/or stiffen a composite and also to aid in static dissipation. It has the same limitations as glass fibers. Carbon fiber can make plastic very stiff.
Minerals such as talc and clay are often used as fillers to reduce the cost or increase the hardness of finished parts. Since they do not shrink as much as resins do when cooled, they can reduce warping.
PTFE (Teflon) and molybdenum disulfide are used to make parts self-lubricating in bearing applications.
Long glass fibers are used like short glass fibers to strengthen and reduce creep, but make the resin much stronger and stiffer. The downside is that they can be particularly challenging to mold parts with thin walls and/or long resin flows.
Aramid (Kevlar) fibers are like less-abrasive glass fibers only not as strong.
Glass beads and mica flakes are used to stiffen a composite and reduce warping and shrinkage. With high loading, they can be challenging to inject.
Stainless steel fibers are used to control EMI (electromagnetic interference) and RFI (radio frequency interference) typically in housings for electronic components. They are more conductive than carbon fiber.
UV inhibitor for outdoor applications.
Static treatments make resins dissipate static.
Surface Finishes for Molded Parts
Surface finish is another important consideration for injection molding since parts are typically cosmetic and intended for end-use production. Keep in mind that textured surface will require more draft than a more polished finish. This is to ensure the part releases from the mold and so that the bead blast can be properly applied to the tool.
These are our surface finish options for thermoplastic and silicone rubber molding
Thermoplastic | Liquid Silicone Rubber |
|
|
In addition to our standard surface finish options, we also offer industry-standard Mold Tech finishes for more complex textures. These can be used to improve grip on a part’s handle or hide cosmetic defects like parting lines. Mold Tech textures can be used to achieve the following effects:
- finishes produced by bead-blasting or polishing the mold surface
- wood or leather-like graining
- pebbled surfaces
- matte, gloss, or hazy finishes, and many others
Post-Processing and Advanced Molding Techniques
Ultrasonic welding: uses a high frequency vibration to generate heat and drive an insert into a part. In more complex molding applications it can also be used to fuse parts together.
Part Marking: There two ways to add images, oftentimes logos, to parts: pad printing and laser engraving.
Pad Printing allows for the addition of color graphics to molded parts at scale. This can be used for adding logos, graphics, and instructions on parts. We offer pad printing for ABS, PC, and ABS/PC resins.
Laser Engraving uses a laser to burn a 2D image onto a part or mold cavity. While color is not an option for laser engraving it is a more cost-effective, faster way to mark plastic parts when compared to pad printing.
Pickouts: Pickouts are inserts that are machined separately from the tooling and placed in the mold before material is injected. They are used to achieve undercuts on interior surfaces. Pickouts are ejected along with the plastic part and placed back in the mold. Using a pickout overcomes many shape and positioning restrictions, but is more costly than sliding shutoffs, or using a side-action.
Steel Core Pins: A steel pin is strong enough to handle the stress of ejection and its surface is smooth enough to release cleanly from the part without draft. There shouldn’t be any cosmetic effect on the resulting part; if there is, it will be inside the hole where it won’t be seen.
Injection Molding Quality Systems
Since companies rely on injection molding to produce thousands, sometimes millions, of parts, quality control is critical.
Scientific Molding: Scientific molding describes a standard process used to develop, optimize, and document the ideal set up process for each injection molding project so that the same process and parameters are followed for every single run. This ensures part consistent for each run of parts.
First Article Inspection (FAI): FAI is a method used to verify that parts are to specification. We take a critical-to-quality approach to injection molding FAIs. Using GD&T, customers can call out the most critical dimensions of their part.
Production Part Approval Process (PPAP): PPAP is a quality inspection process typically used in the automotive industry. The method is used to verify that a production process can reliably produce a quality part repeatedly. There are 18 components to a PPAP inspection that range from customer approval to sample part production.
ISO 13485: ISO 13485 is the standard for medical industry. This includes design qualification (DQ), operational qualification (OQ), and performance qualification (PQ).
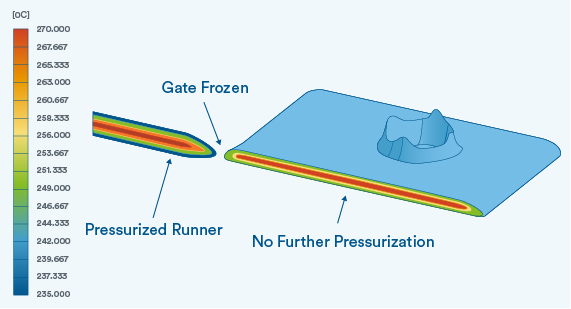