Plastic Manufacturing: Common Processes and Materials
Learn how to select the best manufacturing process and material for your custom plastic parts
Imagine there are no plastics. It’s one of those imponderables if you were born any time after the early 20th century. That’s when the first synthetic plastic was developed by Belgian Leo Baekeland. Bakelite was the start of the revolution we live in today.
Unlike 1907, now we have hundreds of different plastics to work with, and they come in powders, solids, pellets, and liquid resins, each of which offer different advantages, depending on what manufacturing technique you use to make your parts. This guide will help you know more about plastics manufacturing, so you can evaluate the processes and learn about the materials you can use as the basis for your parts.
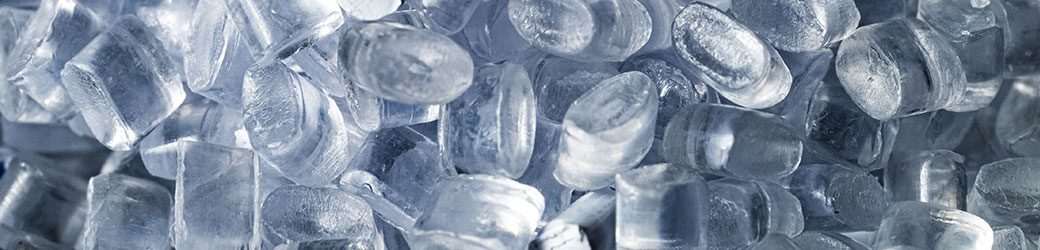
Plastic Materials: Pellets, Resins, and Powders
Choosing the right material for your parts is critical to their functionality and longevity. Beyond pellets, resins, and powders, it also makes sense to mention solid-form plastics, which come in blocks and cylinders, for use in CNC machining. More about those later.
There are two types of plastics: thermosets and thermoplastics.
You can always remember the difference because the end-form of a thermoset part is, well, set. You can’t melt it and use the goo to reform another part. It’s one and done. Generally, thermoset plastics require less heat and pressure to form, but also have high heat and chemical resistance. On the downside, they tend to be non-recyclable.
Thermoplastics are the opposite; while they are built the same way, typically they can be returned to a liquid form and remolded. That said, you do need more heat and pressure to form them into parts. The properties of the parts you get will vary depending on the material you’ve chosen.
Both plastics can be found across the manufacturing ecosystem taking unique journeys to final part based on your chosen service line. If you’re designing medical parts, you have a lot of requirements to adhere to, so you may want to explore some of the more popular plastic materials at a deeper level.
Starting with 3D printing, stereolithography (SLA) uses an ultraviolet laser to cure thermoset resin. When using selective laser sintering (SLS), thermoset and thermoplastic powders are solidified with the zap of a laser. Multi Jet Fusion (MJF) sprays fusing/detailing agents just like an inkjet printer to build out parts layer-by-layer, setting layers of powder as the build progresses.
Injection molding, for example, is more straightforward. You will work with either thermoset or thermoplastic pellets, which are melted into liquid resin so they can easily flow into, and through, a mold. The service also boasts the largest library of plastics. Common materials include:
Comparing Injection Molding Materials
Resin Type | Benefits |
---|---|
POM or Acetal (Polyoxymethylene) | Tough, stiff, hard, and strong. Good lubricity and resistance to hydrocarbons and organic solvents. Good elasticity, slippery. Low creep. Great fatigue properties. |
PMMA or Acrylic (Polymethyl Methacrylate) | Good optical properties, high gloss, scratch resistant. Low shrink, less sink in geometries with thin and thick sections. |
ABS (Acrylonitrile butadiene styrene) | Tough, impact- and chemical-resistant, low shrink, high dimensional stability, inexpensive |
HDPE (High-density polyethylene) | Tough, impact- and chemical-resistant, high shrink, low dimensional stability, inexpensive, density less than water (floats) |
PC (Polycarbonate) | Strong, extremely impact resistant, low shrink, good dimensional stability, and heat resistance, accepts high cosmetic finishes well |
ABS/PC | Strength, heat and low-temperature resistance, improved processing, |
PP (Polypropylene) | Inexpensive, higher impact resistance in some grades, PP homopolymer can be brittle in cold. Wear resistant, flexible with high elongation. Resistant to acids and bases. Density less than water (floats) |
Polystyrene (PS) | High optic clarity, good electric insulator |
PEEK (Polyether Ether Ketone) | High-temperature, high-performance, flame retardant; excellent strength and dimensional stability, good chemical resistance |
PEI or Ultem (Polyetherimide) | High-temperature, high-performance, flame retardant, excellent strength and dimensional stability, good chemical resistance. |
PPSU (Polyphenylsulfone) | High-temperature tolerance, dimensionally stable, high toughness. Resistance to radiation sterilization, as well as alkalis and weak acids |
PA (Aliphatic Polyamides) | Wide variety. High strength and temperature tolerance when reinforced. Chemically resistant except to strong bases or acids |
PPA (Semi-aromatic Polyamides) | Less susceptible to moisture than aliphatic polyamides |
PBT (Polybutylene Terephthalate) | Good electrical properties for power components and works well for automotive applications. Moderate to high strength depending on glass fill. Unfilled grades are tough and flexible. Good resistance to fuels, oils, fats, and many solvents. Doesn’t absorb flavors. Low creep. |
PET (Polyethylene Terephtalate) | Similar to PBT, but stiffer and higher melting point |
LCP | Very easy flowing, good chemical resistance, high upper use temp, good electrical properties, low thermal expansion |
PPO | Good electrical insulator, hot water- and steam- resistance |
PPS | Very good chemical resistance, high upper use temp, great electrical properties |
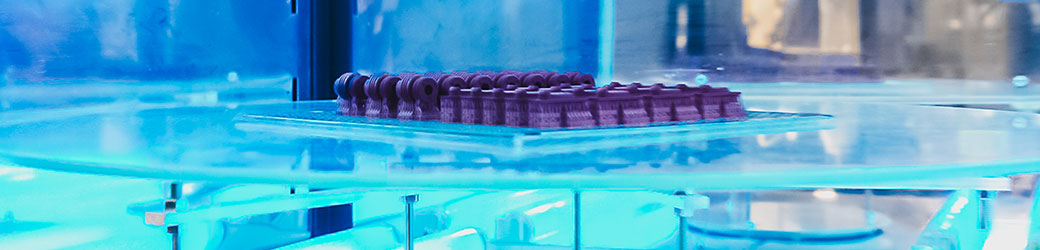
Plastic Manufacturing Processes
Let’s take a look at the primary ways you can manufacture plastic parts, starting with a high-level overview.
Plastic Manufacturing Processes at a Glance
Process | Description | Works Best For |
---|---|---|
Injection Molding | Metal molds are milled, then filled with liquid resin that is cooled to form final parts | Large volumes of parts with simple geometries |
CNC Machining | A subtractive manufacturing process that cuts away plastics from a solid block or cylinder using either a mill or lathe | Widely used in aerospace, medical, automotive industries for its ability to rapidly manufacture precise parts in production-grade materials |
3D Printing | Depending on the technology used, parts are built by fusing, sintering, or extruding material layer-by-layer | Low volume, rapid prototyping and production parts with complex geometries for aerospace, medical, automotive, and other large industry sectors |
Extrusion | Applies pressure and heat to softened material, then pushes it through a specially designed die. Think pasta or Play-Doh®! | Long, continuous parts with the same geometry and hollow parts. |
Blow Molding | A hollow tube of molten plastic (parison) is placed in a mold and injected with compressed air, forcing it to conform to the mold shape, and making it hollow | Bottles, fuel tanks, IV bags, drums, air ducts |
Rotational Molding | Plastic powder is placed inside a mold, which is rotated inside an oven, forcing the plastic to melt and adhere to the mold shape | Tanks, storage containers, traffic cones, medical waste containers |
Thermoforming | Plastic film is heated and compressed in a multi-part mold. Vacuum is used to pull the material against the mold to conform to the desired shape | Food packaging, cosmetics, clamshell packs, trim panels, electronics. |
Now let’s take a closer look at each process.
Injection Molding
Process
At its most basic level, injection molding is all about melting plastics and elastomers and squeezing them through passageways to fill a mold in the shape of a part or group of parts. Once the mold has filled, the material cools and parts are ejected.
The process sounds simple but requires careful design for a smooth journey. Potential pitfalls include:
- Short shots: Lack of material, insufficient flow rates, or poor design can lead to a partially unfilled cavity.
- Insufficient draft: Parts need to angle outward to simplify removal from the tooling without damage.
- Sink: Wide areas of material that cool slower in the middle, creating a sunken appearance.
- Shrink: The outside edges get pulled in by the middle.
- Minimum thickness: Design elements still have to consider the limitations of the mold to support material flow without breaking those potentially critical geometries.
Interested in learning more? Protolabs provides helpful details on common DFM advisories and how to avoid them.
Common Materials
Protolabs offers more than 90 different plastics with our injection molding service, including both thermosets and thermoplastics, plus another 26 elastomers/liquid silicone rubber (LSR) products. Here’s a quick table to fill you in on a few of the uses for some of our most common molding materials.
Standard/commercial materials | ABS, PC, ABS/PC, PP, acetal, acrylic, HDPE, LDPE |
Engineering materials | Polyamides (nylons), LCP, PPS/PPE, PBT blends |
High temperature materials | PEEK, Ultem (PEI) |
LSR | Elastosil, Silastic, Dow Corning |
Thermoplastic elastomers |
There are quite a few alternatives for plastic injection molding that you can explore in this guide. |
Applications and Key Benefits
The combination of a huge selection of materials and injection molding’s established track record as a manufacturing method means you can use this process for most any parts. It excels in high-volume production and cost-effectiveness. Injection molding also offers precision and repeatability. Once you’ve figured out the best parameters to make certain parts, you’ll generally get consistent and desirable output. Best of all, it lets you work with a wide range of materials that run the gamut from thermosets and thermoplastics to elastomers. Here are some examples of common materials/industry pairings.
Electronics/enclosures | ABS/PC/ABS-PC, acrylic, PPE+PS |
Automotive | Polyamides (nylons), LCP, PP, TPEs (thermoplastic elastomers), ABS, PS, polyethylenes (HD & LD), PC, acrylic, PBT |
Medical | PC, LSR, PEEK, PSU, PEI |
Ideal Geometry
Remember that your aim is to design a mold that allows for smooth, consistent, and complete flow of materials throughout, as well as to have parts that avoid the issues noted above in the process section. Incorporate the following for best results:
- Radii Using internal radii can help with flow because sharp corners are difficult to fill.
- Draft Use 1-degree of draft per inch of depth to ensure easy removal of your parts.
- Shutoff angles Maintaining a 3-degree shutoff between mold components will lengthen mold life.
With injection molding, it’s also important to consider the placement of parting lines—the location where the A and B sides of the mold come together. If cosmetic appearance is important to you, think carefully about where that line will appear.
Check out this great resource for best practices in injection molding design.
CNC Machining
Process
CNC machining is a subtractive process, meaning that you create parts by cutting away material from a block or cylinder with incredible accuracy to reveal desired geometries. It’s that speed—from quote to part—that we’re known for. What makes our process especially fast is the digital thread, which turns CAD models into G-code (a way to tell the mills and lathes how to remove material accurately and quickly). We also use a fixed set of endmills and cutters. Tool changes take a lot of time, so by offering the most common cutters and stocking them in the standard set of endmills, we speed tooling changes.
Common variations within CNC machining include milling plastics via both 3- and 5-axis machines, as well as turning. While 3-axis mills can cut along the X, Y, and Z planes, 5-axis adds two rotary axes (A and B). Those two additional axes allow for manufacture of more complex shapes and greater efficiency. On the downside, 5-axis is more expensive and requires higher levels of training to operate. Turning combines both lathe and mill capabilities to machine parts with cylindrical features from metal rod stock.
Common Materials
The most popular plastics by far are POM (acetal) options, specifically POM-H (homopolymer) and POM-C (copolymer). Those plastics are known for their durability, friction resistance (they are self-lubricating), corrosion resistance, and dimensional stability. These properties make POM great for demanding mechanical applications, particularly in the aerospace, automotive, and even medical industries.
ABS and polycarbonate (PC) are close behind in popularity due to their versatility across manufacturing and design worlds. ABS is known for its all-purpose balance between flexibility and stiffness, as well as relative cost effectiveness. On the other hand, PC edges out ABS in terms of flexibility but not rigidity, along with better heat deflection performance. Both are commonly used for enclosure applications given their overall toughness and cosmetic appeal. These materials are favored by medical and consumer electronics industries.
Need extreme high-performance plastic? For challenging environments, consider PEEK or Ultem (PEI). Both are used in very demanding applications, particularly when heat resistance, corrosion resistance, and extreme strength are required. Aerospace, medical, automotive, energy, and even electronics Industries often leverage these materials.
Applications and Key Benefits
CNC machining is well-known for its precision and efficiency. It uses computer-controlled tools to cut and shape a wide range of materials, including metals, plastics—even wood and foam! This translates to several key benefits:
- Unmatched accuracy and repeatability: CNC machines can produce parts with incredible detail and consistency, even if they are based on complex designs. It’s the go-to technology for applications demanding tight tolerances and consistent performance.
- Increased efficiency and speed: The key is automation. Automation eliminates manual labor and allows for continuous operation, significantly boosting production rates. Plus, minimal setup time between different parts further optimizes the process, especially with a defined toolset. When paired with the power of digital manufacturing, you can move from quoted CAD file to completed part in as fast as a day.
- Reduced waste and cost: Precise cutting minimizes material waste, leading to cost savings. Also, as mentioned above, the ability to produce complex parts without manual intervention reduces labor costs.
CNC machining is used to manufacture parts across a huge variety of industries. It's used in everything from intricate medical devices and aerospace components to everyday items like phone casings and car parts. It's also crucial for rapid prototyping, allowing designers to quickly test and iterate on their ideas.
Ideal Geometry
For machined parts, simpler is always better. Less means greater part strength and lower pricing. Avoiding undercut features, particularly for our automated quick-turn service, is always beneficial.
Keeping features on the same machining axes can simplify the machining process itself and potentially save you money. Not surprisingly, only cutting two sides of a block is much more straightforward than features requiring additional side-setups.
Wall thickness is one of the more specifically overlooked elements, too, especially when you increase an unsupported wall/rib in height/depth. There is no hard rule but keeping a 1:3 width-to-height ratio can help avoid feature bending or breaking.
3D Printing
Process
There are a few processes that fall under the heading of 3D printing (or more formally, additive manufacturing) for plastics. Each offers designers and engineers specialized capabilities worth considering, depending on your application.
Stereolithography (SLA) uses an ultraviolet laser that draws on the surface of liquid thermoset resin, creating thin layers until parts are fully formed. SLA is often used to create parts that require detailed feature resolution and smooth surfaces for cosmetic prototyping.
Selective Laser Sintering (SLS) machines make each layer of part geometry by using a laser to melt a bed of nylon-based powder based on the desired geometries. After each layer is fused, a roller moves across the bed to distribute another thin coating of powder. This process is repeated layer-by-layer until the build is complete. SLS yields parts that are stiff, durable, and chemically resistant.
Multi Jet Fusion (MJF) selectively applies fusing and detailing agents across a bed of nylon powder, similar to an inkjet printer. Heating elements fuse the material together in thousands of layers into a solid functional component. Final parts have quality surface finishes, fine feature resolution, and more consistent mechanical properties when compared to processes like SLS.
Carbon Digital Light Synthesis (Carbon DLS) technology produces parts through a photochemical process that balances light and oxygen, projecting light into a reservoir of UV-curable resin. As a sequence of UV images is projected, the part solidifies. Carbon creates plastic parts (often made of ABS or polypropylene) with excellent mechanical properties and surface finish.
PolyJet works by spraying small droplets of liquid photopolymer from multiple jets onto a build platform. These are cured in layers to form elastomeric parts commonly used for prototyping and creating flexible parts.
Common Materials
ABS, polycarbonate, nylon, polypropylene, TPU, and True Silicone are all often used for both prototyping and production components. As it’s a very popular service, people often have questions about what material to use. This guide can help you choose, but here’s a shortcut chart to get you started. Note that some variants of each of these materials may have additional positive attributes:
Material | Properties |
ABS-like | General purpose, strength |
Carbon | Flame resistance |
Ceramic-like | Strength, stiffness, temperature resistance |
Digital Photopolymer | Flexibility |
PA11, PA12 (nylon) | Durability, dimensional stability |
PC-like | Transparency, stiffness |
Polypropylene | Flexibility, chemical resistance, dimensional stability |
PP-like | Flexibility, transparency, durability |
TPU | Flexibility, impact resistance, wear resistance |
True Silicone | Temperature resistance, wear resistance, flexibility, moisture resistance |
Applications and Key Benefits
Over the last decade, 3D printing has become a popular manufacturing process for most industries. Now, companies large and small rely on it to create custom parts for applications in sectors such as aerospace, automotive, electronics, and medical.
Additive manufacturing can be used for both rapid prototyping and end-use production. Some typical printed parts include engine components, fuel injectors, heat exchangers, housings, enclosures, jigs, fixtures, medical devices, and surgical instrumentation. If you can think of the design, there’s a good chance it can be 3D-printed. It almost goes without saying that 3D printing is perfect for unique and complex geometries.
Ideal Geometry
Designing parts with printability in mind is key. At Protolabs, our automated design analysis on CAD models highlights features that can be optimized for manufacturability. Here are a handful of helpful 3D printing design guidelines to keep in mind. Note: These are all dependent on material, technology, and resolution, so reference our Design for 3D Printing Toolkit for specific guidelines.
- Although it varies based on the plastic material, our minimum wall thickness recommendation starts at 0.003 in. (0.076mm).
- Small holes or gaps need to be at least 0.020 in. (0.508mm).
- Maximum Part Sizes: Both technology and material used will dictate the size of the part.
- Inaccessible Surfaces: Avoid areas that are difficult to access, clean, and finish.
- Protolabs pro tip: consider light-weighting designs whenever possible to reduce material usage and overall cost. Learn more about how to cut costs here.
Extrusion
Process
Extrusion creates objects that have a consistent cross-sectional profile by forcing molten material through a die. The shape of the die defines the shape of part. The material you use can be anything that can be turned into a viscous fluid as long as it is soft and pliable.
Heating also ensures that thermoplastics flow through the die before cooling. The heated material sits in a barrel wherein more heat is applied and a screw in the barrel compresses and forces the material through the die. You’re left with a length of the desired shape flowing out. Once that cools a bit, it’s safe to use cutters to cut the length into individual pieces. From a manufacturing point of view, temperature, pressure, screw speed, and die design are all critical parameters.
Common Materials
Plastics such as PE, PP, PVC, PS, and ABS are among the most popular. Extrusion of these plastics, for example, make up the plumbing in a modern house and even your tube of toothpaste.
Applications and Key Benefits
Extrusion’s versatility enables the production of diverse shapes. It’s a cost-effective process in that tooling tends to be basic and pretty much all the material you create will turn into high-volume, consistent, and functional end-use parts. Many industries use extruded parts, including construction, plastics, automotive, packaging, aerospace, and medical devices.
Ideal Geometry
The best part designs for extrusion consider three basic concepts: uniform wall thickness, simple features, and smaller products. Remember that the goal is for material to flow effortlessly with the shove of a screw to move it along. If the die is distorted or rough, that will limit the flow of material. Eliminating sharp corners (adding radii) and adding draft angles will also help flow.
Blow Molding
Process
A first stage in blow molding is often extrusion of material into a cylindrical shape called a parison, which is cut to the desired length of your final product. If it helps, you can head back one section for a refresher on extrusion.
Two halves of a mold surround the parison while it is still warm and compressed air blows through it via a blow pin. The force of the air pins the parison against the mold, creating the desired shape. An air- or water-cooling process follows to solidify the part while still in the mold. Once cooled, the mold is opened and the part is complete.
Common Materials
Look at the recycling number stamped onto the bottom of any of the plastic containers in your house and you’re likely to find one of these: PE (including cousin materials LDPE and HDPE), PP, PET, PVC, PC, and PETG. If recyclability is a concern, PET (#1) and HDPE (#2) are accepted most anywhere, and many jurisdictions also accept PP (#5). However, PVC (#3) and PS (#6) are not easily recyclable. Ultimately, your choice will be based on whether you need transparency, rigidity, chemical resistance, and affordability.
Applications and Key Benefits
Blow molding is a cost-effective and efficient way to produce lightweight, hollow parts, especially via high-volume production. Popular applications include bottles, toys, sporting equipment, and fundamentally anything that can hold liquid. The low tooling costs and minimal material usage/waste are major benefits of blow molding.
Ideal Geometry
As mentioned before, the entire process is about building hollow parts. By maintaining even wall thickness, you can avoid issues with warping and sink marks. Ever bought a bottle of soda and had the bottle tear open on one side? Probably not—because someone did their job.
Adding draft angles will prolong the life of the mold, and corner radii ensure that the chosen material completely covers the mold’s interior, including ribs and reinforcements. Also, don’t forget to carefully place parting lines to lessen visible seams and the dreaded scourge of flash (oozing of material where the two halves of the mold meet).
Rotational Molding
Process
Also known as rotomolding or rotational casting, this is another process that makes hollow parts. In this case, the mold is heated to melt a predetermined amount of plastic powder or liquid. The novel spin with this process is just that—the mold is spun to distribute the melted material using centripetal force. As it spins, the material adheres to the interior of the mold with uniform wall thickness, forming the hollow part. The mold is cooled and the part solidifies before it is carefully removed.
Common Materials
A number of thermoplastics are ideal for this process, including PE, HDPE, LDPE, LLDPE, XLPE, PP, PVC, Nylon (Polyamide), and PET. As with blow molding, the decision will come down to application, as these plastics vary in terms of chemical resistance, flame retardance, thermal stability, translucency, affordability, and even UV stability, especially if the part will be in the sun a lot (kids’ playground equipment, etc.)
Applications and Key Benefits
Rotational molding makes strong parts with uniform wall thickness. Assuming the mold has been well-designed, you’ll have minimal material waste and fewer defects. And speaking of those molds, you can design complex shapes and even undercuts.
Again, the key is a well-designed mold that ensures the molten plastic can find its way into every nook and cranny. As with other molding processes, this is a good way to make hollow parts, including playground equipment, storage tanks, and for applications that require chemical- and corrosion-resistance.
Ideal Geometry
Your aim with these parts is to create a mold that incorporates draft angles and corner radii. These will help ease removal of your part from the mold and make it safer, too. Whenever possible, avoid undercuts and other complex features.
Also consider how to hide those scratchy parting lines caused by flash. Add vents and holes from which air can escape from between the part and mold—that’ll help improve your part. If you use inserts or plan for assemblies, carefully consider that the combination of parts will fit together easily.
Thermoforming
Process
Thermoforming starts with a sheet of thermoplastic that is warmed then placed atop a mold that is the exact opposite of the shape you want the plastic to become. Vacuum forming pulls the sheet down to ensure a clean mold before the part is cooled and removed. Pressure forming is often used as an extra step for an even tighter mold when fine details or surface textures need to translate to the final part.
Common Materials
With this process, you need thermoplastics to get your desired shape to solidify once the plastic has cooled. Favorite materials include: PS, PE, PVC, PP, ABS, PC, PET, and PETG. Consider chemical-, heat-, and impact-resistance, along with stiffness and durability. If recycling is important, PET and PETG are recyclable almost everywhere, and some places will accept PVC, PP, and PS.
Applications and Key Benefits
You see thermoformed products all over the place in a variety of sizes. Think of the takeout meal with the ubiquitous clear cover or the plastic container at the hardware store that holds ten screws when you only needed one—all thermoformed. One of the greatest aspects of thermoforming is how rapidly it forms hundreds of parts quickly and consistently. Even automobile components are often stamped out. It allows for quick design modifications and even somewhat complex shapes. There are a variety of surface finish options, too. Thermoforming really shines when you need parts in quantity.
Ideal Geometry
It probably seems like a broken record at this point but remember to design with uniform wall thickness and add corner radii and draft angles to ensure that the plastic covers the mold completely and ejects without issue. What is new to consider is that you are stretching a sheet of plastic to form these parts, so you need to use plastic that is longer and wider than your basic part dimensions—called the draw ratio.

How is Quality Measured in Plastic Parts?
So, you’re about to approve a quote from your manufacturer and you want to know how that company determines whether the part is successful. Some of it starts with knowing the inherent qualities of the plastic you’ve chosen but ensuring that geometries follow your specification is equally important. We’ll cover both.
Note that not every method will be used as this is more of an overview of possible measurements. Options will also differ based on your chosen service line. With 3D printing for example, Protolabs offers both digital inspections and mechanical testing, if desired. We check for defects like surface imperfections, warping, and any issues that may affect performance, safety, or durability.
Material Properties
Let’s start with how we measure key material characteristics. Impact testing is as it sounds—dropping a mass onto the material until it shatters. Chemical resistance measures a material’s reaction to a variety of caustic materials or solvents, like strong acids and bases. Another method is to test out the material’s melting point. Finally, you can try to stretch the material under controlled conditions for tensile testing, providing statistics on part strength and elongation.
Dimensional Accuracy
Have a finished part? Let’s start with the basics: calipers and gauges. These handheld tools are great for checking one or two dimensions but can be time consuming for much more than that. Vision systems use cameras and image processing software to visually examine parts and flag any deviations from specs. Many companies opt for coordinate measuring machines (CMMs). These use lasers or probes to measure critical dimensions and compare those to the original 3D CAD model.
Visual Inspection
Sometimes, nothing is better than a good pair of eyes. Doing a visual once-over with trained inspectors will give you a pretty good sense if the part suffers from surface defects like warpage, voids, cracks, or discoloration.
Non-Destructive Testing
When you need to delve into the inside of your part, you can use options not unlike tests you may encounter at a doctor’s office. Computed tomography (CT scans), X-ray radiography, and ultrasonic testing can all reveal potential flaws inside a part, without affecting the material.
Other Options
If meeting specs in all of your parts is important to you, consider requesting a first article inspection so you can feel confident that the systems are delivering parts to your expectations. Also, with injection molding, Protolabs offers a process called CTQ (critical-to-quality), wherein you can specify in your 3D CAD model what the most important geometries are in your part. It’s a great way to signal what could be showstoppers if tolerances cannot be met.
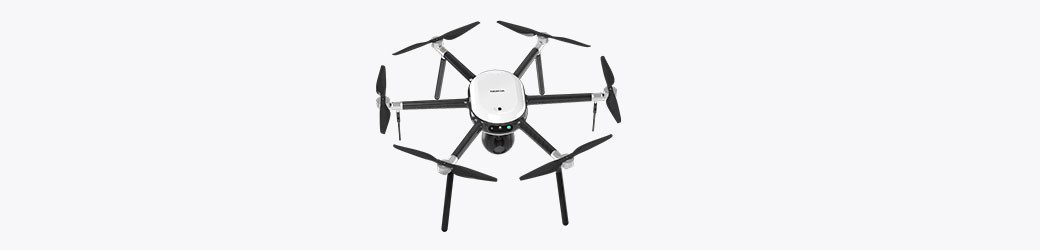
Real-World Examples of Plastic Design Challenges
Plastics can be used for a huge variety of applications and some of them can be surprisingly rugged, as you’ll see from these examples of companies that have worked with Protolabs’ factory services.
3D Printing: BrailleDoodle
For blind and low-vision kids, one of the most important things they can do is learn how to read Braille, but only 10% learn this critical skill. The TouchPad Pro Foundation set out to produce a functional, affordable prototype for a tablet designed to help these students learn Braille anywhere. Users can drag a magnetic stylus over its surface, pulling small metal balls in the holes to the surface in various patterns to create Braille letters, figures, or images. Plastic springs beneath the balls hold them firmly in place until the user pushes them down again. The design required 3,000 plastic springs to hold metallic balls in place at just the right tension.
With Protolabs, BrailleDoodle used ABS-like plastic and stereolithography (SLA) to build an affordable, accurate prototype that adhered to their exacting specifications. SLA was a sensible choice because of its high-resolution printing ability and fine surface finish, allowing the balls and springs to work as designed. Their engineers used design for additive manufacturing (DFAM) capabilities built into our quoting system to refine their designs in preparation for printing.
The nonprofit now has an impressive prototype to demonstrate at conventions and meetings. The Foundation has already received 500 orders for the product and plans to move to production soon.
Injection Molding: Nokia
When it comes to drones, light-weighting components is critical. Plus, when it comes to security, technology and design must work together effortlessly to achieve the highest certification standards. Nokia came to Protolabs to build 19 different part designs for casing, structural elements, and a gasket to get their high-tech, enterprise-level surveillance system approved.
When working within the 5G cellular spectrum, antennas and other devices must be placed thoughtfully to avoid issues with signal attenuation. Metal parts can affect radio transmission and reduce signal quality, too, so plastic was the way to go. Plus, high-capacity batteries can pose a safety risk as they can be highly flammable. The choice of plastic and elements of design were part of those considerations. In the end, we used a particular polycarbonate thermoplastic to lessen weight and simplify molding. It’s strength and resistance to fracturing was perfect for this product.
The molded components not only protected the elements inside the drone and provided the necessary aerodynamics required for a high-performance unmanned aerial vehicle (UAV), but they were also intrinsic to the strength of the drone, ultimately holding the device together.
CNC Machining: Whoop
Now a major player in the fitness tech industry, we started working with Whoop in their startup days. The Whoop sensor is worn like a watch and measures multiple health-based metrics, which are then sent to a mobile device for data collection, storage, and analysis. Fundamentally, wearable devices can only be wearable if they are lightweight and physically small. The plastic components Whoop needed had challenging geometries and some of their smallest ones came very close to our size limitations for CNC machined parts of 0.25 in. x 0.25 in. (6.35mm x 6.35mm) with a nominal thickness of 0.040 in. (1.02mm).
Using our advanced quoting system, Whoop engineers were able to manipulate parts and materials to ensure adherence to design for manufacturability (DFM) and cost efficiency. Plus, their parts came to them in days, rather than weeks or months, saving precious time in a very competitive marketplace.
In all, Whoop saved two weeks in initial CAD model design and overall prototyping. This allowed them to follow an exceptionally ambitious schedule.
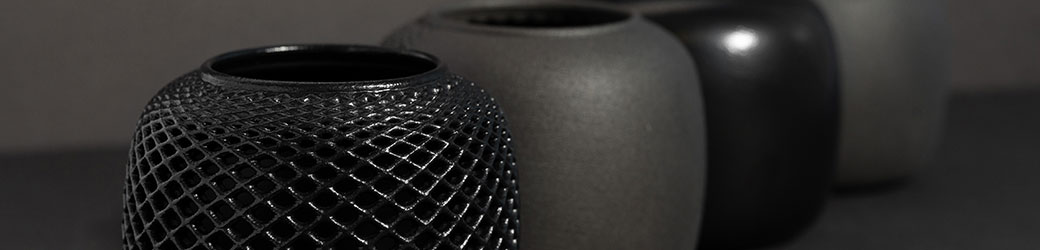
Future Trends and Developments
Because plastics manufacturing is always improving, what we see as trends here will likely go mainstream in record time. The future is now in terms of sustainability, design strategies, and manufacturing techniques.
Emergence of Bioplastics
A major focus across the manufacturing and product design world is how the industry can make plastics more environmentally friendly with innovation in bioplastics.
In general, for manufacturing, injection molding has been ahead of the curve when it comes to material variety and biocompatible plastics. A big reason for that is that injection molding is inherently production, rather than prototype driven. When the scientists and engineers developing these materials look at where these bioplastics can have the biggest impact, it’s in replacing traditional plastics produced at high volumes.
Also, there are different processing considerations with different manufacturing methods. There has been considerable effort put towards creating bioplastics that can run on existing injection molding equipment with minimally disruptive adjustments made to accommodate those materials. Unfortunately, those considerations change drastically when you start looking at producing a block of bioplastic material and expect it to withstand the strain of the CNC machining process. There’s an element of cost as well. Currently, bioplastics can be very expensive compared to the more mass-produced standard plastics and that cost affects both engineers who want to design bioplastic parts and the manufacturers considering working with those same materials.
While barriers exist for widespread adoption, the future looks bright. Every year there are new and exciting developments that make concepts like machining bioplastics more realistic and viable. Recently, a material sciences lab at the University of Washington used blue-green algae to create a new biocompatible material that is reportedly machinable, along with several other beneficial properties. We listen to where our customers want to go and bioplastic materials will only grow in popularity and demand as they become more proven, reliable, and ubiquitous.
Where is Plastics Manufacturing Heading?
In terms of plastics innovation, 3D printing is leading the way in exciting developments rapidly expanding its applications. The emergence of secondary processes that strengthen parts and improving aesthetics is one area of note. Vapor smoothing, for example, uses chemical vapors to eliminate roughness on certain SLS or MJF printed parts, resulting in a smooth, glossy finish like an injection molded part.
Light-weighting is another new direction for plastics manufacturing design. Combining the strength of certain plastics with strategic design can yield parts that require less material, less fuel for shipping, and other advantages. This is most prevalent in 3D printing, where the incorporation of lattice structures is producing stronger parts while shedding weight.
Automated quality control speeds validation and delivery of parts after manufacturing. The days of calipers are slowly ending as we see more robust versions of coordinate-measuring machines (CMM) introduced to the manufacturing floor. Increased automation in quality control is both producing better parts and allowing manufacturers to get those parts out the door faster.
Strategic nearshoring has become a popular way to avoid inventory shortages. Many companies are finding local or regional suppliers to handle their plastics manufacturing as an adjunct, or a replacement, for sending their work offshore. The sourcing strategy may have increased in popularity during the COVID-19 pandemic, but ongoing benefits may mean it is here to stay.
Pursuing Plastics
There’s a reason why we’re surrounded by plastics: They do the job. They are versatile, affordable, and functional, whether you’re building a car or medical equipment. The key is to do the research to match design, materials, and processes so you get the parts you expect. New plastic and elastomer materials emerge every year and you might be surprised by the breadth of what they can do for you.